A significant challenge in graphene fabrication is the production of large, suspended graphene layers. This issue applies to any kind of graphene-based device and is particularly critical when the fabrication of closed cells is required, for example in the fabrication of large, sealed cells for in-operando XPS or TEM investigation, or the fabrication of Golay cell-like bolometers. Since in the quasi-totality of working protocols, graphene transfer is performed in a liquid environment, the following drying step involves the generation of surface tension forces, that are strong enough to break the ultrathin graphene layer and cannot be avoided when sealed cells are fabricated. An alternative route involves the sealing in a second step, which however poses severe requirements in handling and alignment when micrometric structures need to be fabricated. We developed a method to fabricate large arrays of independent micrometric cells, sealed with an ultrathin membrane of single-layer Graphene coupled with a nanometric film of evaporated titanium. The system was then sealed from the bottom with a self-aligned silicone-rubber sealing cork, achieving a yield larger than 95% for the fabrication of closed, sealed microcells, and arrays as large as 10 mm2.
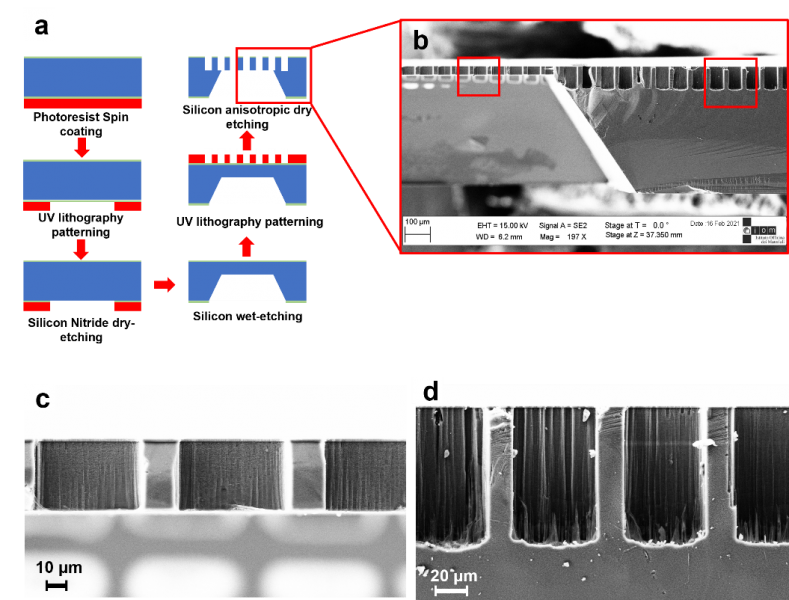
Figure 1 a) schematic illustration of the microfabrication protocols for the grid b) Section view of the grid microfabricated through the silicon wafer. c) particular of open (passing through) and d) closed cells respectively. The closed-cell part of the sample was created when the dry-etching Bosch-like process micromachined the pattern outside of the silicon wet-etched window.
The micromachined grids were fabricated with UV lithography and dry etching techniques applied on a silicon wafer as illustrated in figure 1. The results of the process were square holes into a silicon window with 50 μm of lateral size and a depth of 30 μm. These grids were then used as substrates for the transfer of the bilayered membranes produced by E-Gun evaporation of 7.5 nm of titanium on commercial monolayer graphene.
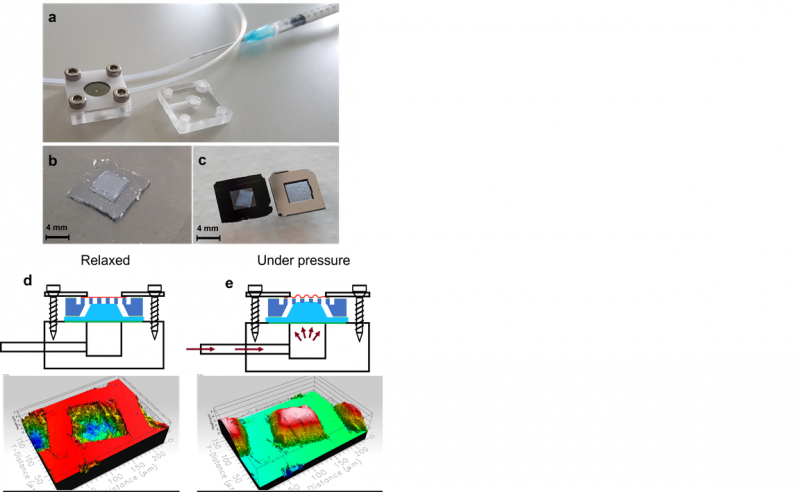
Figure 2 a) Photo of the holder with a sample clamped and the PMMA support. b) Silicone cork used to apply pressure to the sealed grid. c) samples frontside with grid partially covered by the membrane (left), and silicon window backside before dry etching (right). d) and e) illustration of the pressure holder before and during the pressure testing of the cork-grid-membrane sample, and below one of the sealed cells responding to 10 kPa of pressure.
After sealing the top of the grid through the membrane wet transfer, the sample was placed on top of a soft silicone rubber cork (Young modulus E<0.8 MPa) shown in figure 2b, which deformed independently into each cell, sealing their bottom. The sealed cells were then tested on a pressurized sample holder to check on their airtightness and measure their response to pressure differences across the membrane. The holder was based on a laser-cut PMMA block with a cavity and a tube connected to a syringe to control the pressure under the sample as shown in figure 2. By increasing the pressure, the silicone cork deforms inside the bottom of the grids increasing the internal pressure of the cells and causing the swelling of the ultrathin membrane on the top. The membranes under up to 20 kPa of pressure reached a measured height of 3.5 μm and responded independently from each other, without inter-leakage between the cells as shown in figure 3.
In this work, our group demonstrated the fabrication and testing of large arrays (up to 16 mm2 in a scalable design) of micro-cells, sealed over the whole array area with freestanding ultrathin membranes on the top, and a detachable and reusable silicone rubber cork on the bottom. The high yield of the process (over 95%) compared to other ultrathin membranes respond in particular to a fundamental requirement for the translation of this technology to the industrial world. Additionally, our design demonstrated the capability of being resealable and reusable by removing the weakly bonded silicon cork and thus allowing for reduced microfabrication time and cost compared to other one-use arrays of sealed cells.
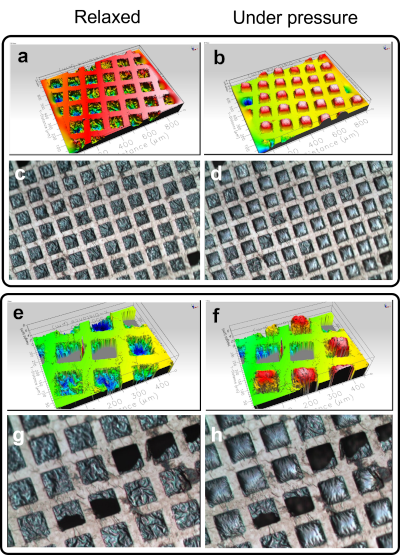
Figure 3 Optical images and profiles of the sealed cells before and under pressure applied. Top, a), c) and b), d) array of cells responding to the pressure presenting the same maximum deflection at atmospheric pressure (relaxed) and under 20 kPa (under pressure). Bottom, e), g) and f), h) cells swelling even in the presence of adjacent damaged membranes, showing the absence of interlinkage between the cells sealed by the cork.
The reusability could also allow changing the sealed material with different gases, liquids, or solids, with different properties such as absorption coefficient for electromagnetic radiation. Thus, the sealed arrays could work as Golay cell-like detectors, with sensitivity depending on the material inserted in the cells and its absorption band. These detectors, compared to the designs present in the literature, would also present larger suspended areas of the membranes, hence improving the efficiency in the detection of longer wavelengths like those of the THz radiation, while using stronger and more reliable membranes compared to the monolayer graphene alone. This work, thus, paves the way for future improvements in the fields of microsensors fabrication as well as all those applications that require large suspended ultrathin membrane.
For more information: https://www.sciencedirect.com/science/article/pii/S259000722200017X